Elements, Heavy Metal, and the Death of Stars






















Where do heavy elements come from? Part Three of Baking an Apple Pie from Scratch (figuratively) examines how stars create heavy elements with more Protons than Iron.
In Part One of this three-part series, we introduced fundamental particles, protons, and neutrons. We saw how primordial nucleosynthesis produced light elements up to Boron [symbol B; 5 Protons + X Neutrons].
In Part Two, we looked at fusion processes inside stars. Stellar nucleosynthesis provided insight into the forming elements up to Iron [symbol Fe; 26 Protons + X Neutrons].
In Part Three, we will examine processes to produce elements with more Protons than Iron.
“The lifetime of a human being is measured by decades, the lifetime of the Sun is a hundred million times longer. Compared to a star, we are like mayflies, fleeting ephemeral creatures who live out their lives in the course of a single day.”
– Carl Sagan, Cosmos
Fusion is Life
Fusion defines the life of a star. A star is born when fusion starts. A star dies when fusion ends. Dying stars release elements back into the universe to birth of the next generation of stars and their planets.
We will look at stars’ life cycles in a future post. If you wish to read independently, I suggest searching for “Main Sequence.” Today’s post focuses primarily on how elements are formed and distributed into space when stars die.
“All the elements other than hydrogen and helium make up just 0.04 percent of the universe. Seen from this perspective, the periodic system appears to be rather insignificant. But the fact remains that we live on the earth… where the relative abundance of elements is quite different.”
― Sam Kean, The Disappearing Spoon: And Other True Tales of Madness, Love, and the History of the World from the Periodic Table of the Elements
Pathways to Heavier Elements
There are two neutron processes which produce most of the elements heavier than Iron. The R-Process is a rapid capture of neutrons. The S-Process is a slow capture of neutrons. The R- and S-Processes either directly form elements or indirectly form them through decay processes.
A few elements (P-nuclei) cannot be formed through the neutron processes. We will refer to these as “P-Processes” (P for proton) for the sake of simplicity in this short post, but this is not technically correct. The “P-process” originally meant a proton capture process. Here we will use it as a shorthand for the synthesis of P-nuclei.


S-Process Element Formation – Slow Cooking
Stars with a mass of 0.6 Solar Mass to ~ 10 Solar Masses form approximately half of the naturally occurring elements above Iron on the periodic table. The S-process is “slow” in a relative manner. It may take seconds to tens of thousands of years, but the key to differentiating is the length of time before additional neutron captures. The S-Process starts with a seed nucleus and captures a neutron. If the result is a stable isotope, then the element remains the same. Additional neutron captures may occur, resulting in an unstable isotope undergoingbeta decay[1]. The extra proton transforms the original element into a new element because elements are defined by the number of protons in their nucleus.
In smaller stars such as our sun, the source of neutrons is provided through a Carbon-13 + Helium-4 (alpha particle) fusion yielding Oxygen-16 + a free neutron. These types of stars will eventually shed their outer layers and expose a white dwarf core.
Larger, more massive stars employ a second s-process to produce free neutrons. Neon-22 + Helium-4 (alpha particle) fuse to form Magnesium-25 and a free neutron. These stars will explode as supernovas.
The upper limit for the s-process (regardless of neutron source) results in Polonium [Po; 84 Protons + X Neutrons], Bismuth [Bi; 83 Protons + X Neutrons] or Lead [Pb; 82 Protons + X Neutrons].
“Here’s a question to give you a sense of scale. Which of the following would be brighter, in terms of the amount of energy delivered to your retina: A supernova, seen from as far away as the Sun is from the Earth, or the detonation of a hydrogen bomb pressed against your eyeball?”
― Randall Munroe, What If?: Serious Scientific Answers to Absurd Hypothetical Questions
R-Process Element Formation – Cooking with a BOOM
The other half of elements heavier than Iron form in a rapid neutron capture process (R-Process). Rapid is relative and defined by neutron capture processes happening before Beta decay can occur. Also, unlike the S-Process, there is no requirement for a seed element such as Iron. While S-Processes generally involve neutron captures every 10 to 100 years, R-Processes may involve 100 neutron captures per second. This requires a high density of free neutrons and exceptional energies.


Where do R-Processes Take Place?
Supernova, Hypernova[2], Quark-nova[3], white dwarf mergers, and neutron star mergers are R-Process sites. R-process elements have also been created on Earth in thermonuclear explosions where Uranium-238 was a seed isotope. Both Einsteinium [99 Protons + X Neutrons] and Fermium [100 Protons + X Neutrons] were discovered in the fallout from thermonuclear devices tested in the 1950s. R-Process elements naturally occurring on Earth include Platinum [Pt; 78 Protons + X Neutrons], Gold [Au; 79 Protons + X Neutrons], and Uranium [U; 92 Protons + X Neutrons].
We have observational evidence for even the most exotic of these collisions, the neutron star merger. A gravitational wave (GW 170817) observed by the LIGO and Virgo detectors on 17 August 2017 captured the merger of two neutron stars. The observation was confirmed by a gamma-ray burst (GRB 170817A) approximately 1.7 seconds after the neutron stars merged. Finally, astronomical telescopes found a short-lived event (AT 2017gfo) in galaxy NGC 4993 11 hours after the gravitational signal. The observations continued for several weeks in the radio to X-ray spectrum and confirmed there was a fast-moving (~ 0.1c or 10% of the speed of light), rapidly-cooling cloud of neutron-rich material. Mathematical models of the merger indicate approximately 16000 Earth masses of R-Process elements formed, including 10 Earth masses of platinum and 10 Earth masses of gold!
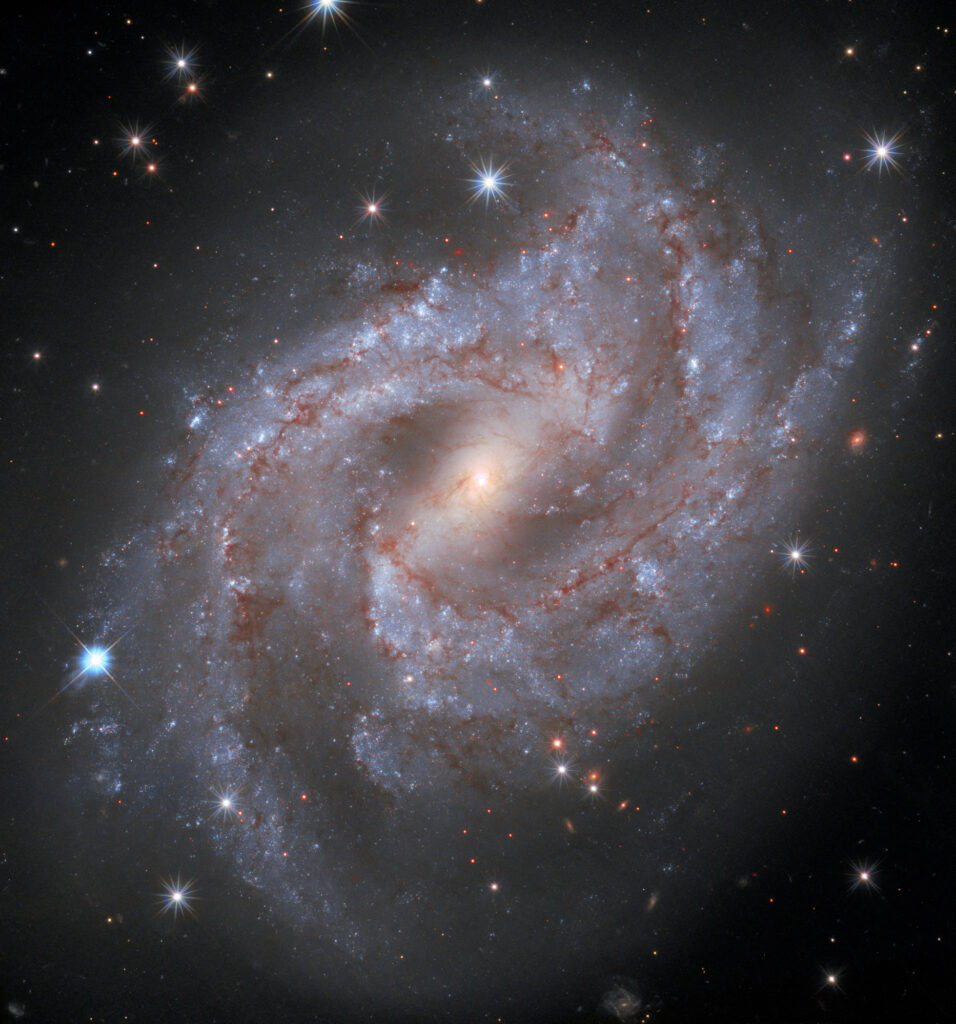
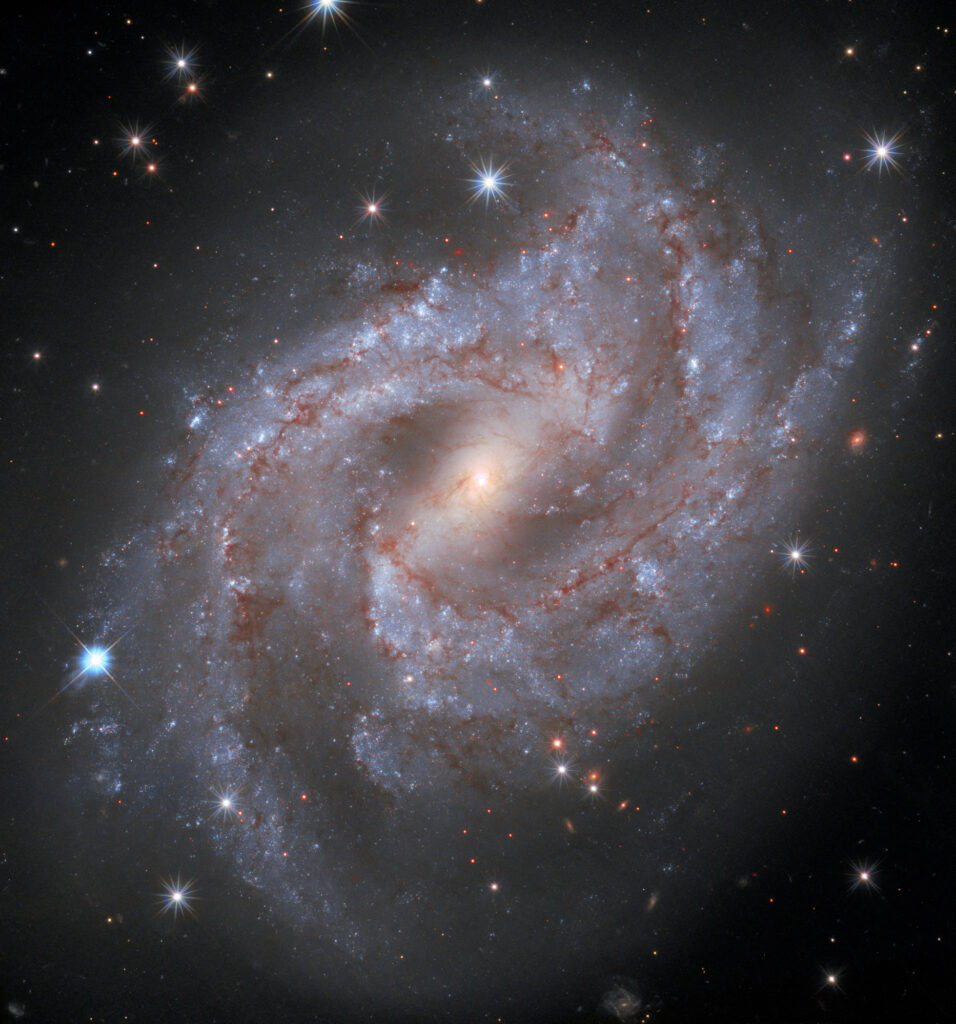
“P-Process” Element Formation
P-Process is no longer an accurate terminology, but in this post, we use it as a shorthand term to group several pathways describing the formation of a small number[4] of elements not known to form through S- or R-processes. These elements are P-nuclei. Examples include Palladium-102 [Pd; 46 Protons + 56 Neutrons], Cadmium-106 [Cd; 48 Protons + 58 Neutrons], and Mercury-196 [Hg; 80 Protons + 116 Neutrons].
The term P-Process originally indicated the formation of elements through Proton capture on stable seed nuclei. Hydrogen is a single proton and readily available in stars. However, the P-Process cannot be responsible for all the P-nuclei.
P-nuclei can also be produced through the Gamma Process. At extremely high temperatures (2-3 Gigakelvins), some S-Process and R-Process nuclei will photodisintegrate by emitting gamma rays. There are additional neutrino-triggered nuclear reactions in core-collapse supernovae which can produce P-nuclei. Finally, a combination of proton captures with Gamma- or Neutrino-Processes can create P-nuclei.
Learn more about elements: The Secret Lives of the Elements
We are the Children of Stars
As we have seen, an apple pie made from scratch requires elements formed in the early universe and nucleosynthesis inside stars.
The oxygen we breathe, the calcium in our bones, the iron in our blood, the silicon in our computer, and all the gold on Earth were formed and released by previous generations of stars.
We take these elements for granted, but their abundances on Earth are atypical for their distribution in the universe. The relative abundances on Earth and in the Solar System give us clues about the star which seeded the cloud the sun formed in.
We are, in a very tangible sense, the children of stars.
Looking Ahead
Now that we have answered some questions about matter and the elements, we have several topics which could be future posts:
- Exploring the Main Sequence stars and their lifecycles
- How stars fade away or die -> Types of Supernovae, Neutron Stars, and Black Holes
- The birth of our solar system
- The Periodic Table
- Element Families
- An Introduction to Chemistry
If Physics, Astronomy, and Chemistry are not your cup of tea, please check back in a few months. There will be other topics I write about, esp. wildlife.
Endnotes
- In beta decay, a neutron becomes a proton and releases an electron and a positron (“anti-electron).
- Hypernovae are supernovae with ejecta having an order of magnitude (10x) times more energy than typical core-collapse supernovae. The ejected nickel mass is high, and ejecta may move up to 0.99c (99% the speed of light). SN1998bw is an example. Hypernovae are associated with long-duration gamma-ray bursts.
- A Quark-nova is hypothetical. The recent supernova SN2006gy may be an example of a Quark-nova. Quark-nova are triggered when a neutron star slows down its spin enough for the extreme pressures to force neutrons to degenerate into constituent quarks.
- The original list of P-Nuclei was only 35 isotopes. In the meantime, several isotopes on the list have been shown to be possible with S- or R-Processes.























