







By Alyssa L. Abbeyn (@ifDiscswereRock) for The Biota Project (@thebiotaproject)
It’s a pretty regular night, my partner and I are doing some extra work tonight since we are leaving early tomorrow to celebrate my birthday. I’m on my computer at the kitchen table and they’re in the other room. Quite suddenly, the house creaks and groans rocking back and forth like a rickety old table with unstable legs. My first thought, “Wow the upstairs neighbors are really stomping around up there, or maybe some furniture fell down or something…” then it hits me, the noise wasn’t from upstairs and it wasn’t just a rumble…the entire house creaked one way and then the other. As this is running through my head my partner rushes into the kitchen and we both look at each other, “Was that an earthquake?!”
Indeed, it was. On October 14th 2019, at 10:33 pm (local time) a magnitude 4.5 earthquake ruptured near Pleasant Hill California (less than 20 miles from Berkeley and Oakland CA, and less than 30 miles from San Francisco, CA). 10 minutes prior to the main 4.5 shock was one 2.5 magnitude foreshock—called a foreshock because it happens before a larger seismic event. In the hour following the main shock there were over 20 aftershocks (all less than magnitude 2.1, so we didn’t feel any more shaking). This is the first earthquake I have ever felt! And, although we were unsure if anything bigger was about to follow, the first thing I did was to navigate to the USGS Earthquake Hazards Program webpage (https://earthquake.usgs.gov/earthquakes/map/).
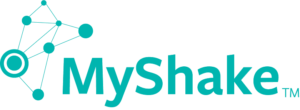
Meanwhile, only one mile away Sarina Patel, a PhD student in the Berkeley Seismology Laboratory, was driving home on a pothole ridden street and didn’t notice anything out of the ordinary. Upon arriving home, to an unusually hectic scene, she was bombarded by questions from her housemates, who had just had a similar experience to mine. Sarina, who has been working on research related to damage identification in buildings after seismic events, knew about the USGS website as well, but also knew that the earliest, fastest, confirmation of an earthquake event is actually Twitter! So, when she arrived home to a chaotic house and a flood of questions without knowing about the earthquake that had just occurred, the first place she went for details was Twitter. Her next move was to check an app on her phone called MyShake (https://myshake.berkeley.edu/).
Earthquake app
The MyShake app (@myshakeapp) was first developed at the Berkeley Seismological Laboratory by Dr. Qingkai Kong, during his PhD, as a citizen science crowdsourcing project for monitoring earthquake hazards. However, the project has expanded and in partnership with the USGS it is now also an earthquake early warning app in the state of California! That week Sarina was on “moment tensor duty”, or more fondly referred to as “pager duty”, because scientists used to be contacted on a pager when a seismic event happened. Being on “duty” means she was one of the seismologists on call to process all the data that comes in about an earthquake and refine the initial automatic computer-estimated magnitude.
On October 14th, the night of the Pleasant Hill earthquake, the early warning part of the MyShake app was due to be released in three days, and so although many people already had the app on their phone, it was still the citizen science-only version of the app. Only the people on the developing team, like Sarina, had the early warning beta version on their phones. The Pleasant Hill earthquake was extremely well-timed and helped the development team test the alert system just in time to release the public version of the early warning system. What luck!
The MyShake app can be downloaded for free and essentially works by monitoring vibrations felt by the phone. It observes three aspects of the shaking (1) the amplitude—how big the wave is, (2) the frequency—how often the waves come, and (3) the cumulative absolute velocity—the speed of the waves. In the beginning, the most difficult problem with building the shaking detection code for the app was figuring out how to tell the difference between shaking from an earthquake, and shaking from something else, for example, a train going by. Well, it turns out earthquakes plot in a distinct location on a graph of the three factors mentioned above (red dots here in a figure from Kong et al., 2016).
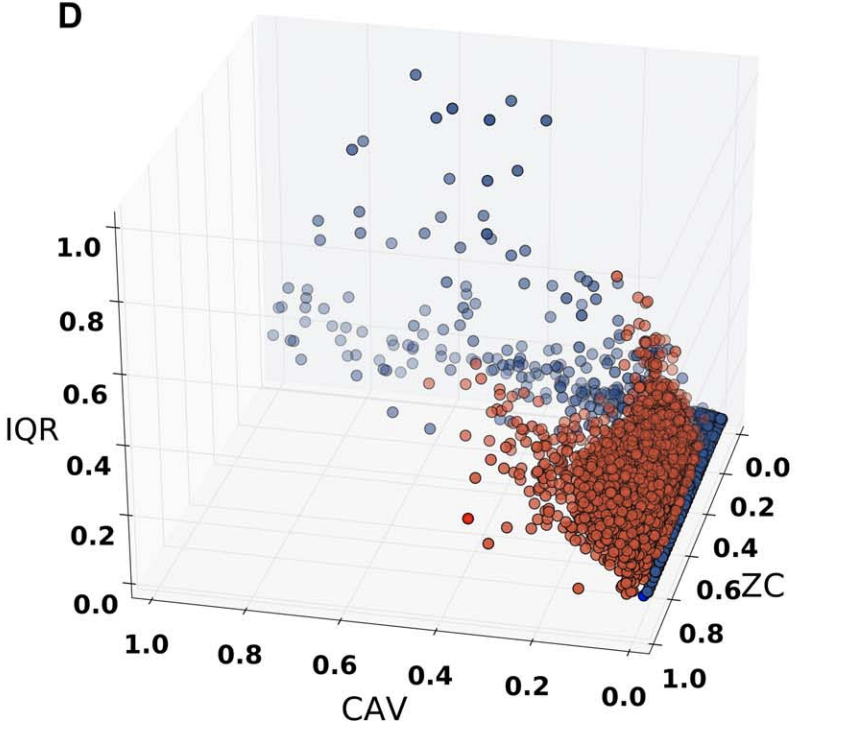
For detecting and monitoring a seismic event all the phones with the MyShake app installed actually work together. If one phone feels shaking that it thinks belongs to an earthquake, the app will find out if other phones are detecting the same thing, and once there are several phones nearby, in the network, that all register the type of shaking expected from an earthquake, the system will register a new seismic event. To find out more about the MyShake app visit the website (https://myshake.berkeley.edu/) or try downloading the app onto your phone and become a citizen scientist to help the Berkeley Seismology Lab gather earthquake information.
Earthquakes: working to build mountains
Earthquakes are sudden short-lived events that sometimes lead to significant amounts of infrastructure damage especially if they occur in areas with large human populations, which is what Sarina studies at UC Berkeley. However, unlike lightening, earthquakes can occur in the same place twice. What I mean by that is that earthquakes occur when part of a fault breaks or slips from a previously locked state, and often only small sections of a fault will experience slip at one time. So, when I say an earthquake can happen in the same place twice it means that the same fault will experience a slip-event tens, to hundreds, to thousands of times.
Every time a fault slips it creates an earthquake and the magnitude of the earthquake relates to the size of the fault area that slips and type of rock material that the fault is breaking. Faults generally move vertically, horizontally, or some combination of the two. You can imagine then, that if one side of a fault slides up by one foot relative to the other side, and this happens 50 times there will be a pretty big difference in the height (or elevation) on each side of the fault. Basically, this process of faults slipping over and over, creating earthquakes over and over is one geologic process that shapes the landscape—the topography on the surface of the Earth! This is how mountains are made! One earthquake that causes shaking for a few seconds is part of a much longer and larger system that involves many earthquakes over thousands to millions of years working to create topographic change on Earth’s surface. The main factor shaping the landscape is time.
Rivers: working to shape mountains
Another key component to shaping the Earth’s surface is erosion. Erosion, essentially the destruction of a feature via physical or chemical processes, is similarly reliant on time. For example, wind can be a strong erosive force that works by picking up small dust and sand particles and battering them against a mountainside, slowly chipping away at the solid rock. Likewise, water plays a huge role in eroding and shaping topography. Small streams that start on the tops of mountains carve channels into the land as the force of the flowing water picks up sand and plants moving the material downhill and out of the way. One stream will join another creating a larger stream that has more power to pull up larger rocks and dig a deeper channel. Joining streams can combine to become large rivers that cut huge canyons over millions of years, like the Grand Canyon. Again, it’s all about time.
The Grand Canyon, AZ. The top from the North rim (left) and the bottom from the valley floor (right) Images taken by Alyssa Abbey in October 2017
Most erosion happening from streams is slow and continuous, as long as there is a source of water like rain or snow melt. However, there are times when, analogous to short-lived large earthquake events, there are short-lived large flood events, that can cause a massive amount of erosion at one time. Such events can cause a rerouting, or change in the location of a stream, they can change the shape and structure of the stream channel, and they can move huge rocks and large amounts of sand and mud, also called sediment. These flood events are often called 100-year flood events, which actually means “a stream has a 1% chance of having an extreme flood event, and ‘extreme’ is different for every place depending on the statistics from that specific location, which are based on historical events”, says Ryan Hummel, project engineer for Ecological Resource Consultants Inc. based in Colorado. Ryan (@wedge1039), who works on stream restoration projects all over the state, was in CO in September of 2013 when the colossal floods occurred near Boulder, CO leaving people stranded either in their homes unable to leave or out and unable to go home.
The 2013 floods were a 50-year event caused by large amounts of rainfall, but they were not predicted to be 100-year events because of the way such events are categorized. The event prediction relates to the amount of rainfall in a 24-hour period, and although there was a lot of rain in Colorado, the 24-hour time period was not notably out of the ordinary. The substantial amount flooding occurred because there was an entire week of constant rain. The small mountain streams with narrow channels only one-foot wide had trouble holding all that water and acted as chutes funneling the water downstream with very high energy, strong enough to wash out culverts—the pipes that carry water under roadways. This powerful flow of water was also able to turn these small streams into rivers five times their original size moving boulder sized rocks down the newly shaped streams. This stream widening and reshaping, a process that might normally take hundreds of years, was accomplished in a matter of days.
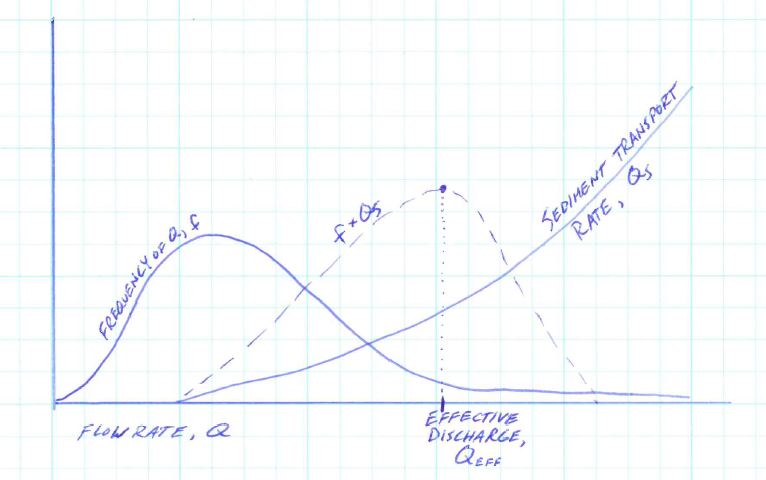
Stream channel reshaping occurs when flow is high enough to transport the sediment making up the channel bed and banks. The higher the flow rate, the more sediment gets moved, meaning the higher the flow rate, the greater amount of erosion that occurs in the channel. The high flows that will transport the most sediment, however, do not occur regularly. The flow rate that will have the most impact on the channel shape is the flow that will move a large amount of sediment, while also occurring on a frequent basis. This is called the effective discharge. When engineers like Ryan begin to make predictions about when or where a channel forming or channel reshaping event might occur, they approximate the effective discharge by multiplying the frequency of each flow event by the sediment transport rate of that flow and plotting the products as a curve (see Ryan’s rendition of this concept by Wolman and Miller, 1960, shown here). The effective discharge is the flow rate at the peak of this curve. Stream restoration consulting companies are often used for the purpose of identifying and preparing for flood events in both mountain streams in the middle of nowhere and streams that flow near places populated by humans.
Other stream restoration projects are sought after for a number of reasons including (a) recreation and tourism, as restoring streams can often help improve fish and wildlife habitats, (b) safety, for instance dealing with flooding events or chemical contamination from waste products like mine tailings, or (c) environmental and ecological rebuilding, such as is needed after a mining or construction operation closes. An example of such an endeavor is the Swan River restoration project in Summit County CO (http://www.co.summit.co.us/Blog.aspx?CID=5), which is working to reestablish a natural, healthy, and vibrant ecological system in the mountains, which used to be an old mining area.
The Swan River Restoration Project, Summit County, CO. Pictures taken to document the restoration, left: 2015, middle: 2016, right: 2017. (to learn more about the restoration visit http://www.co.summit.co.us/Blog.aspx?CID=5)
Building and restoring streams is a much faster process than how change occurs in nature, but companies like Ecological Resource Consultants Inc. work to make all of their modifications reflect what streams would do in nature, often using historical imagery as a guide. Essentially, this means a lot of narrowing and deepening of existing channels, making a pool on one side and a shallow bank on the other, or adding sinuosity—the snake-like winding shape, because most urban channels are extremely straight and flat. In fact, next time you go out walking by a trickling stream in the woods, shuffling through a dried up wash in the desert, or hiking up a rocky mountain slope, see if you can identify the different natural characters of streams and compare that to the diverted water systems running through towns and cities.
To learn more about the altering landscapes around you follow The Biota Project this month on twitter and Instagram as we explore various forms of change across different amounts of time.
—Alyssa Abbey is a postdoctoral fellow at UC Berkeley doing research on mountain building by understanding processes behind fault growth and erosion. Alyssa received her PhD from the University of Michigan, studying continental rifting. In her free time, she enjoys hiking, bike touring, playing Ultimate Frisbee, and rock climbing.
References
Kong, Q., Allen, R. M., Schreier, L., & Kwon, Y. W. (2016). MyShake: A smartphone seismic network for earthquake early warning and beyond. Science advances, 2(2), e1501055.
Wolman, M. G. and Miller, J. P. Magnitude of Frequency of Forces in Geomorphic Processes. The Journal of Geology. Vol. 88. No. 1. January 1960.







