The neurobiology of anorexia






















Anorexia’s connection to dopamine and serotonin is the focus of a new study on how brain signals affect eating behaviors.
Anorexia is an eating disorder that typically involves food restriction (i.e., not eating or under-eating), weight loss, and body dysmorphia (meaning: perceiving one’s body to be larger than it really is). Anorexia is a serious mental health challenge that is often chronic and debilitating. Many people with anorexia end up suffering from stomach ulcers, bone loss, and hormonal problems. Terrifyingly, anorexia is the deadliest psychiatric disorder: one percent of the affected patients die because of severe weight loss over their lifetime. The Center for Discovery reports that this death rate is 12 times higher than the combined death rate from any other causes of death for females between 15 and 25 years old. Anorexia affects both men and women, and while anorexia can begin at any point during one’s lifetime, it most often starts in the mid-teens (ages 13-15). People with anorexia are typically treated with psychotherapy and antidepressants, but these treatment strategies are often not effective in weight-restoration or better body-image, often leading to no recovery or relapses. Furthermore, researchers still do not understand how and why some people suffer from anorexia. Xing Cai and fellow researchers at the Children’s Nutrition Research Center in Houston published a paper in the journal Nature Neuroscience that has revealed new insights into some of the biological mechanisms driving the onset and maintenance of anorexia.
Prior to this study by Cai and colleagues, researchers reported a genetic link between anorexia and two neurotransmitters: dopamine and serotonin. Some of those reports have found that certain polymorphisms (meaning a variation in a gene’s sequence: the genetic sequence differs from the normal sequence, but it is not a mutation) in the genetic sequence for one of the dopamine receptors leads to a higher risk of anorexia. There are several biological markers that link abnormal dopamine and serotonin neurotransmission to anorexia. For example, individuals with anorexia have reduced dopaminergic and increased serotonergic metabolites (metabolites are byproducts of neurotransmitters after they start getting broken down); increasing serotonin levels in mice or humans induces anorexic behavior (i.e., reducing food intake). Yet many studies have found that serotonin is important for regulating normal feeding behavior. Cai’s research team extends on these findings by showing that abnormal signaling of serotonin and dopamine can drive the onset of anorexic behavior in mice.
Research by Cai and colleagues encompassed many experiments to make these novel conclusions. Here, we will summarize the main results from their research report.
A specific type of Dopamine receptor in the Dorsal Raphe Nucleus brain region drives anorexia in mice
Previous work from other laboratories has found that feeding behavior is regulated by dopamine releasing neurons that reside in a brain region called the Ventral Tegmental Area (VTA) (Figure 1A). These dopamine releasing neurons have axons that project to another brain region called the Dorsal Raphe Nucleus (DRN), where they can modify the activity of the DRN-residing serotonin releasing neurons. If the dopamine neurons only display weak levels of activity they will activate a subset of dopamine receptors, called the D2 receptors, that inhibit the activity of the DRN-residing serotonin neurons. This inhibition ultimately leads to overeating in mice. In contrast, if the dopamine neurons in the VTA display stronger levels of activity, they will activate another subset of dopamine receptors, called the D1 receptors, that lead to activation of serotonin neurons, and ultimately food restriction and anorexia. In mice without any known traumatic experiences or genetic differences (we refer to these as “wild-type” mice), feeding behavior is guided by glucose and metabolic homeostasis. When individuals have low levels of energy, hormones are released that lead to a weak activation of VTA-residing dopamine neurons, reduced activity of DRN-located serotonin neurons, and ultimately food intake. It is hypothesized that this brain circuit drives motivation for food intake, and perhaps also food appetite. On the other hand, if you have just had a large filling meal, other types of hormones are released that lead to stronger activation of VTA-dopamine neurons, increased serotonin activation in the DRN, and ultimately reduced food intake (or food restriction). As you go through your day these brain circuits will be activated sequentially to ensure that you maintain a healthy energy level, without overconsumption or starvation. Cai’s research team used electrophysiological techniques to demonstrate how this circuit works, and by using optogenetics, they could control whether a hungry mouse would eat or not. When they stimulated the dopamine neurons with low levels of activity it led to food intake (Figure 1A, see the arrow named “Weak (tonic) activity”). Conversely, if they increased the dopamine neurons’ stimulation in the VTA the otherwise hungry mouse would stop eating (Figure 1A, see the arrow named “Stronger (burst) activity”).
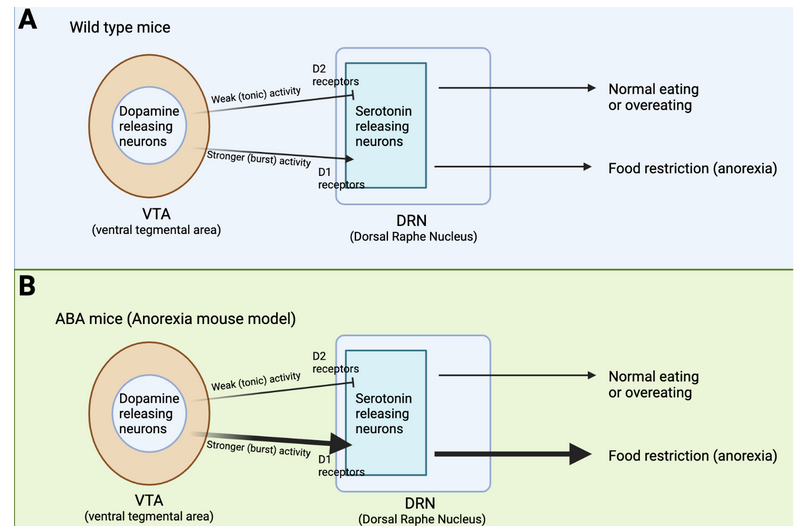
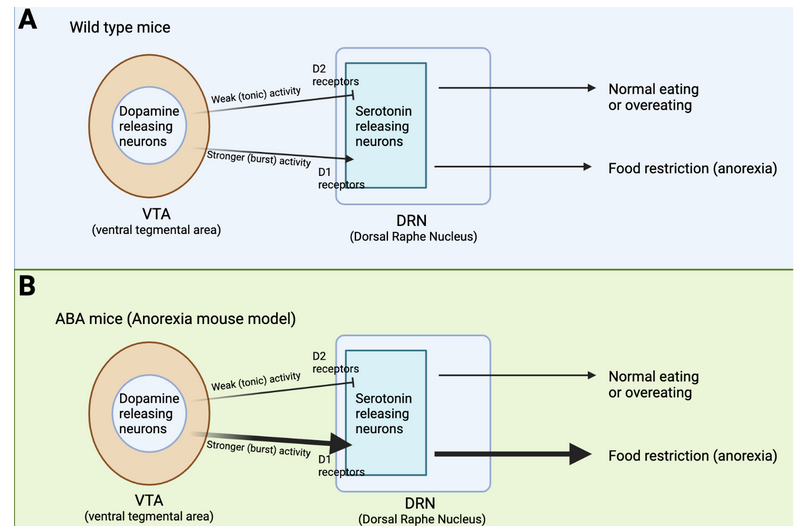
What is the difference between low and strong levels of stimulation? Neurons are activated by electrical stimulation, which can be anywhere from less than one Hz to over hundreds of Hz. Neurons will display different types of activation depending on how much (or how little) they were stimulated. A 2 Hz stimulation will lead to a different activation pattern than a 20 Hz stimulation. That’s exactly what Cai’s team found. A low stimulation with 2 Hz led to weak activity of the dopamine neurons in the VTA which led to activation of D2 receptors, and ultimately inhibited serotonin neurons. In contrast, a high stimulation with 20 Hz electricity led to strong activation of VTA-residing dopamine neurons, which activated D1 receptors and led to serotonin neuron activity.
How does this brain circuit tie into anorexia? It is difficult to model anorexia in a mouse, and while it cannot be done perfectly (as is true for so many other diseases as well!), one mouse model, called the activity-based anorexia (ABA) paradigm, captures aspects of anorexic symptomatology (Figure 2A and B). These mice are not genetically modified in any way. In fact, their anorexic behavior is only triggered by two things: 1. Chronic food restriction (i.e., they have access to some food, but never enough to be satisfied), and 2. Constant access to a running wheel. Note that these mice are not forced to be active on the running wheel, however, shortly after being in a cage with reduced food and running wheel access, the mice will start overexercising. Overexercising is a common (but not always present) symptom in anorexia, and this mouse model may capture the biology of a certain subset of anorexic patients that overexercise and undereat. ABA mice display increased activity in the dopamine axons projecting from VTA to DRN, as well as increased activity in the serotonin neurons in the DRN (Figure 1B). This increase in serotonin activity was mediated by D1 receptors. Cai’s team measured this activity increase by using a technique called Calcium-imaging. When neurons are active there is a surge in calcium levels inside of their somas (also called cell-bodies) and axons. Calcium-imaging therefore gives researchers the ability to understand what is happening in the mouse brain while the mouse is awake. Curiously, if mice were subjected to acute food starvation (24 hours without food), it actually led to a decrease in serotonin neuron activity in the DRN, ultimately driving food intake. These results tell us that dopamine and serotonin neurons display different activity after acute versus chronic food restriction. In other words, chronic food restriction and overexercise leads to D1-receptor-dependent activation of DRN-residing serotonin neurons. This circuit drives continued food restriction (and overexercise) even when facing reduced energy levels. Cai’s team did not figure out whether this change is led by the overexercise in the running wheel or simply a product of lack of food (or maybe a combination of both), but it does suggest that changing the activity of this brain circuit could prevent or reduce the anorexic symptoms in the ABA mice.


Reducing D1 receptor activation reduces anorexic symptoms in ABA mice
Cai’s team used genetic tools to produce a new type of mouse that lacked D1 receptor expression in the DRN. This means that even if VTA-residing neurons displayed strong activity, they would not be able to activate D1 receptors in the DRN, and therefore not enable increased activation of DRN-residing serotonin neurons. Indeed, when these mice were subjected to chronic food restriction and constant access to running wheels, they did not end up overexercising (Figure 2C). In fact, these mice ate more and lost less weight than what normally happens to mice in the ABA paradigm (Figure 3). Moreover, these genetically modified mice displayed reduced serotonin neuron activation in the DRN, consistent with the idea that the “food restriction” brain circuit was not activated by the ABA paradigm. These results suggest that reducing D1 receptor expression in the DRN could be a new way of treating and preventing anorexia.
However, to treat anorexia in humans we cannot modify their genome. Cai’s team therefore decided to test if using a pharmacological drug to block D1 receptor activation was equally as successful in reducing anorexia symptoms of ABA mice. Indeed, when mice received the drug during the ABA paradigm, they ate more and ran less. Just like the genetically modified mice, pharmacological blockade of D1 receptor activation reduced anorexic symptoms. These results suggest that D1 receptors are important for driving the onset of anorexia, and indirectly, they tell us that the other type of dopamine receptor, the D2 receptor, prevents anorexia. Indeed, Cai’s team found that if they produced mice that lacked D2 receptors (rather than reducing D1 receptor expression) in serotonin neurons of the DRN, the mice overexercised in the running wheel and reduced their food intake. These results emphasize that D2 receptors are important for preventing anorexic behaviors. It is noteworthy that previous research found polymorphisms, or gene variations, in the D2 receptors of people with anorexia. In the context of these results by Cai’s team, these polymorphisms may have reduced D2 receptors from being appropriately activated, leading to increased susceptibility to anorexia when overexercising.
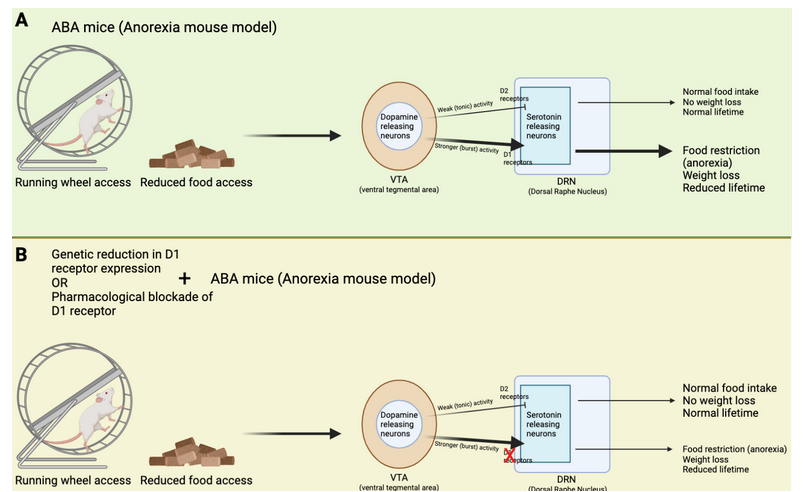
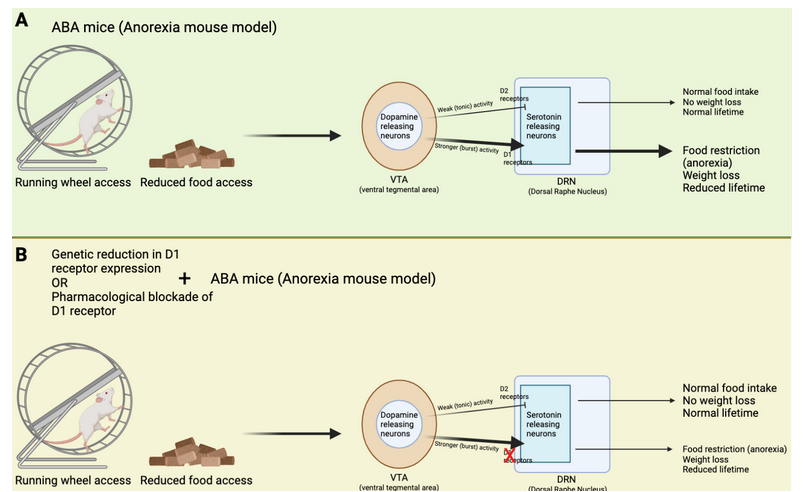
In conclusion: a novel treatment for anorexia?
Cai’s research team uncovers a novel insight into the neurobiology of one form of anorexia that is characterized by overexercising combined with under-eating. The research is critical in shaping our approach to treatment of anorexia, which at present is highly limited. The research tells us of strategies that can be used to prevent the onset (and subsequent maintenance) of anorexia upon access to overexercise. Perhaps blocking D1 receptors in teenagers who are about to start competitive sports could prevent the onset of anorexia in these individuals. It will also be important to test if using drugs to block D1 receptors could help treat individuals who have already suffered from anorexia for a long time.
References
Arcelus, J., Mitchell, A. J., Wales, J., Nielsen, S. (2011). Mortality rates in patients with anorexia nervosa and other eating disorders: A meta-analysis of 36 studies. Archives of General Psychiatry, 68(7): 724–731. http://www.doi.org/10.1001/archgenpsychiatry.2011.74
Cai, X., Liu, H., Feng, B., Yu, M., He, Y., Liu, H., Liang, C., Yang, Y., Tu, L., Zhang, N., Wang, L., Yin, N., Han, J., Yan, Z., Wang, C., Xu, P., Wu, Q., Tong, Q., He, Y., & Xu, Y. (2022). A D2 to D1 shift in dopaminergic inputs to midbrain 5-HT neurons causes anorexia in mice. Nature Neuroscience, 25(5), 646–658. https://doi.org/10.1038/s41593-022-01062-0
Center for Discovery. (2021). Five statistics of anorexia nervosa and its consequences. https://centerfordiscovery.com/blog/statistics-behind-anorexia/
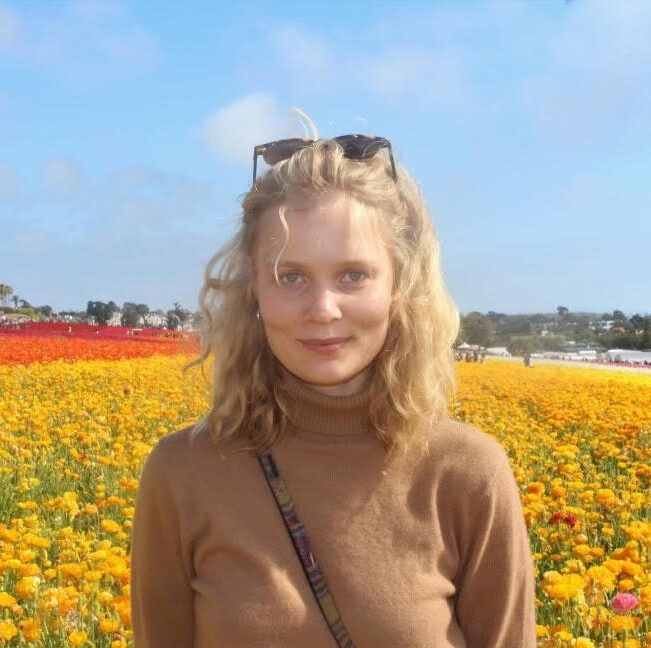
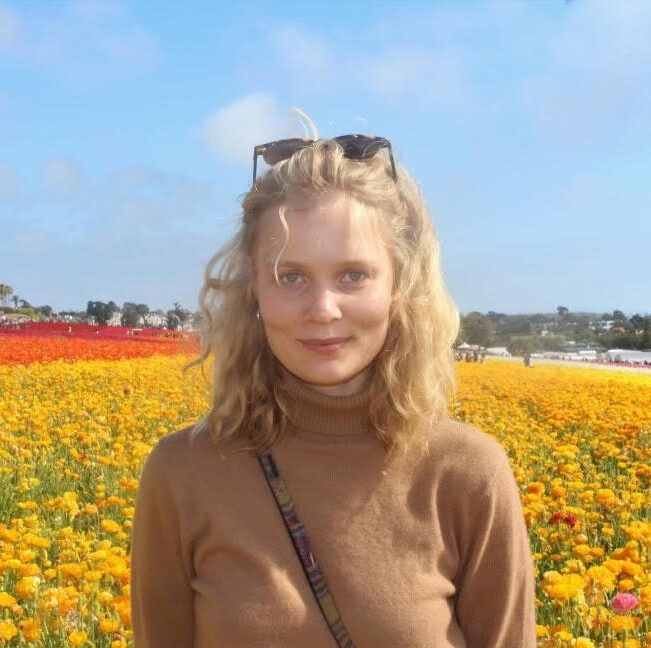
About the Author
Pernille Bülow has a PhD in Neuroscience and currently works as a Product Specialist on neurophysiology at iMotions. She is passionate about understanding and questioning the mind-body relationship. In her spare time, Pernille practices fencing, horse riding, performing aerial acrobatics and reads lots of fiction. Find her on LinkedIn: https://www.linkedin.com/in/pernille-bülow.
The information contained in this article is for informational purposes only and is not intended as health or medical advice. Always consult a physician or other qualified health provider regarding any questions you may have about a medical condition or health objectives.























