Nests, Neurons, and the Evolution of Behavior






















Evolution is a beautiful process. We tend to appreciate its elegance most when looking backward into deep time– to our distant ancestors, dinosaurs, and the evolution of behavior.
Evolution is a beautiful thing. We tend to appreciate its elegance and creative productivity most when looking backward into deep time: in our distant ancestors, in dinosaurs, and in entire strange lineages that rose and fell before humanity. To most of us, evolution is a thing that happened so long ago that it has no practical relevance to our lives today. We see its results in the bodies of living things and the relationships among them—and these results, in all their wonder, seem static, like final products at the end of an eons-long assembly line.
But evolution is still happening. It is working its magic all around us: in the emergence of hundreds of domestic subspecies from the wolf, in the sprawling diversity of tropical warblers, and in the uncanny wonder of gorillas and chimpanzees that now suffer under competition from another ape. And it is working even closer to home as well, within our own species. It has given rise to the unique sets of instructions for building and regulating the proteins that lie hidden within our cells, and to the phenotypes these proteins produce. Selection, whether natural or otherwise, requires variation to work. Whenever the cold mechanism of selection favors genetic variants for their causal effect on fitness, evolution is happening.
Just as the process of evolution has produced a docile dog from a wild wolf, in humans too it cannot have stopped below the brain. If we assume that the present time is an unexceptional one in the genetic history of our species, individual differences in behavior must exist because they are the raw material on which natural selection works. And in a species whose success is dictated more by behavior than by canine teeth or body size, it should come as no surprise that our actions, choices, and abilities are affected by heritable variation. This vast reservoir of genetic diversity is in turn the result of millions of years of mutation and natural selection acting on our planet’s dominant species, a snapshot in geologic time of a frothing, active gene pool.
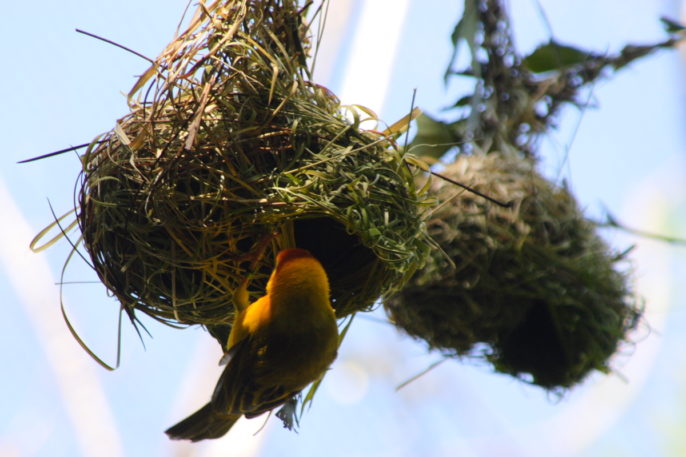
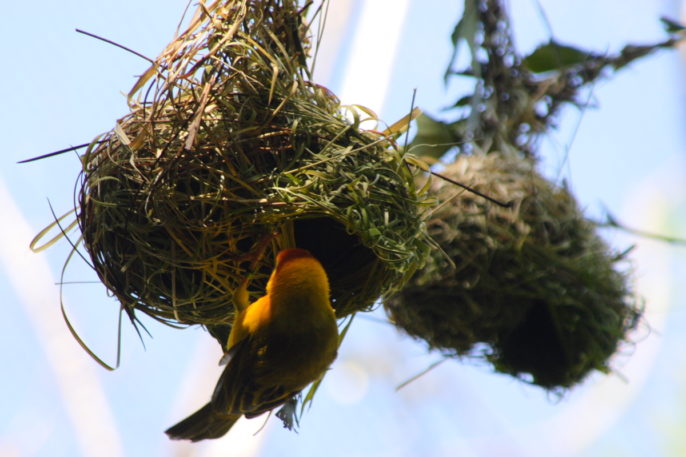
Understanding Behavior in the Genomic Age
In 1982, the evolutionary biologist Richard Dawkins suggested in his book The Extended Phenotype that its titular concept, normally defined as the totality of an organism’s observable traits, should be extended to all the effects its genome has on its environment. Limiting the influence of genes to an animal’s anatomy is arbitrary, Dawkins claimed. “I find that my kind of paradigm examples are things like beaver dams and birds’ nests, where I’m trying to shake people into realizing that you could have a ‘gene for’ a certain shape of birds’ nest, just as surely as you could have a certain shape of beak. You could selectively breed for nest shape. You could do a Mendelian experiment. You could do an artificial selection experiment. You could take a hundred generations to breed weaver birds that make nests of a different shape.”
There is surely no single gene “for” nest-building, just as there is no gene “for” any complex human behavior. But consider what is meant by the idea that the shape of a bird’s nest is affected by its genes. The act of building is influenced by a great many things: the builder’s ability to notice and find certain materials, the sensitivity to the weight and balance of how they are laid, a tradeoff between spending less time and energy or having greater protection for the eggs, and so on. In reality a number of different genes influence each of these qualities, and together they allow a bird to form a nest whose shape is heritable in the same way that beak shape is heritable.
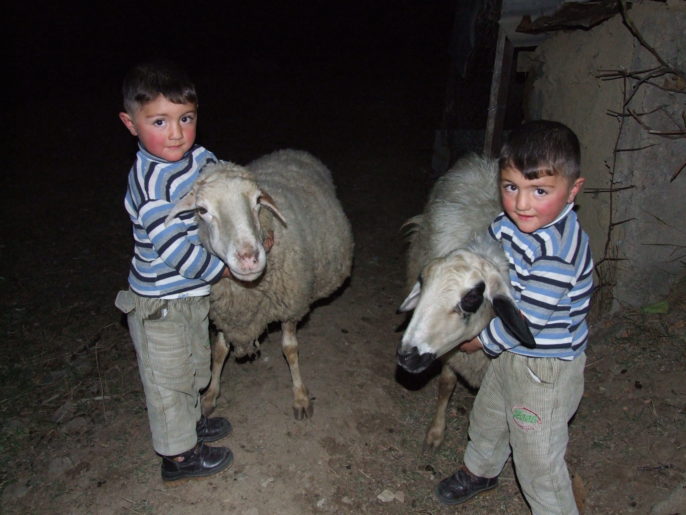
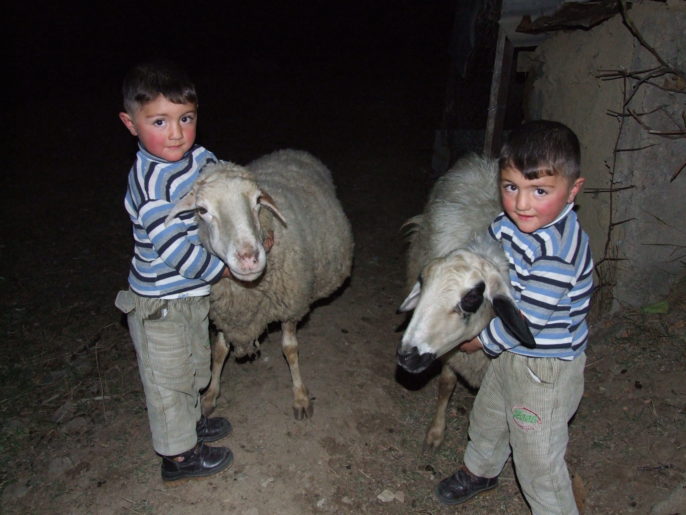
A combination of twin studies and psychometric testing has repeatedly shown us that most measurable human behaviors have some kind of heritable component as well. Of particular interest has been intelligence, as measured by an IQ score or standardized tests. Over a century’s worth of data has converged on a few key points: Intelligence is highly heritable; it is expressed via a great many genetic variants; and it is predictive of certain life outcomes. Taken together, these suggest that our evolutionary history has generated a set of genetic variants that can have relevant influence over our environment—the extended phenotype come to life.
The Genius of Genome-Wide Association
Only recently has the field of behavioral genetics starting giving us the tools to examine some of this variance, and its phenotypic expression, in much higher resolution. One such method, genome-wide complex trait analysis (GCTA), estimates the chance of genetic similarity across hundreds of thousands of variants for each pair of individuals and relates this genetic similarity to phenotypic similarity for a particular trait. This analysis uses genetic variants called single-nucleotide polymorphisms (SNPs) that differ between individuals at a specific nucleotide. GCTA has consistently revealed a strong genetic component to cognitive ability and to socioeconomic status that corroborates twin studies, while making fewer assumptions.
Another powerful and promising tool in modern behavior genetics is genome-wide association (GWA), which is used to identify specific genetic correlates of observable traits. This method works by overlaying the genomic data from a large number of participants, which covers a wide range of common genetic variants, and looking for associations with phenotypic data from the participants. For example, if one version of a defined string of DNA shows up in participants with schizophrenia at a rate far above the population average, this string of DNA can be thought of as “associated” with schizophrenia.
The first successful GWA study, which found two genetic variants associated with macular degeneration, was performed in 2005. Since then, over 200 diseases and traits have been studied with this technique, and nearly 4,000 SNPs have been isolated. The GWA design has been used to track down SNPs that influence traits and susceptibilities such as longevity, schizophrenia, Alzheimer’s, various cancers, and more. The beauty of the genome-wide association technique is that it works just as nature does in all its elegance—by detecting, as natural selection does, whether a given allele is correlated with the phenotype.
The methodology of the GWA study has undergone a lot of evolution over the past decade, and it has not been without its critics, but its modern iteration has extraordinary predictive power. While ever far from explaining the full breadth of human behavior, this technique is beginning to look like a powerful tool in the quest to unpack the genetic correlates of real-world behavioral outcomes. A starting point was the landmark study by Cornelius Rietveld and colleagues which appeared in a 2013 issue of Science. Using genome-wide association in over 100,000 participants, the team found three SNPs that were highly replicable predictors of not only years of education but also—in an independent sample of some 24,000 participants—cognitive performance, as captured by a general factor (g) extracted from a diverse battery of tests. Together these SNPs accounted for a very small portion of the overall variance in educational attainment, but their genome-wide significance and reproducibility in a later independent study seems to confirm what twin researchers have always suspected: that cognitive performance and its many correlates, while strongly heritable, are influenced by a vast number of genes, each of small effect. Intelligence, like height, is a polygenic trait.
Evolution: From Genotype to Phenotype
The structure of a weaver’s nest is subject to countless environmental influences: the shape of the branches on which it is built; the stressors of wind, weather, and predators; available resources for building; and the random chance implicit in any macrophysical process. But the environment can only shape what already exists in the bird’s mind. In this way, a set of genetic variants controls the proteins that unfold in development, which control the organization of neurons and brain structures, which then control the preferences and abilities that lead, eventually, to a nest. At any point in the chain, environment and random chance can influence its shape. But all the causal agents, including the genes, are indispensable if a full understanding of the outcome of evolution is the goal.
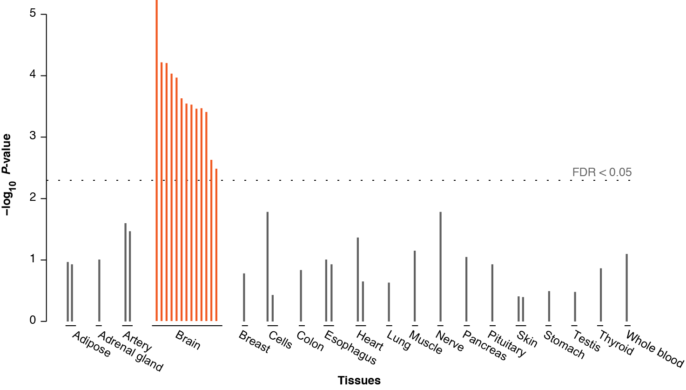
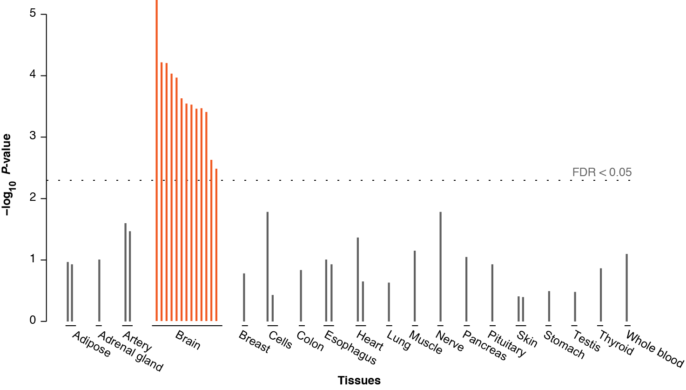
In this year’s May 26 issue of Nature, research carried out by the Social Science Genetic Association Consortium (SSGAC) has built upon Rietveld’s 2013 study to link together part of our own behavioral chain. Using genome-wide association techniques, the team has identified 74 loci associated with years of educational attainment, a well-known predicted outcome of psychometric intelligence. This study replicates many of the SNPs found to influence educational attainment in the previous meta-analysis, and again, the same SNPs that predict variance in IQ also predict years in school. Remarkably, protein-coding regions in these loci are also strongly expressed in the neural tissue of the prenatal brain. This is borne out by the extent of overlap between the 74 loci associated with educational attainment and known clusters of genes that regulate neural development. Significantly enriched clusters include those for neuron migration, axon projection, dendritic sprouting, and synaptic plasticity.
The current estimation for total genetic contribution to variance in educational attainment is at least 20 percent. In the new study, the polygenic scores derived from these loci account for only about 3.2 percent of this variance so far, and each genetic locus on its own has a very small effect. But because of the vast size of the sample, the chance of even such a faint signal being random noise is essentially zero. Additionally, a follow-up study published July 19 found that these polygenic scores explained a whopping 15 percent of the variance in educational achievement scores at age 16—the strongest prediction of polygenic scores for a behavioral trait so far.
And so, by tracking variants in human genome evolution through neural development, to cognitive ability, and onward to years of schooling and even to achievement scores, behavioral geneticists have elegantly unearthed some of the proverbial genes “for” the human equivalent of nest building itself.
The Strongest Link in the Chain
Few people have a problem with ascribing evolution or a genetic basis to the height or eye color of humans, or to the nests and dams of birds and beavers. Yet to many, the idea that a few nucleotides can raise the boat for those who have them—even by a mere few weeks of education—will feel deeply uncomfortable. In the social sciences, one particularly salient paradigm to explain certain behavioral differences is privilege theory, the view that success in the real world is determined only by the unfair advantage society affords some people over others, rather than by innate abilities. Ascribing a genetic underpinning to some of these life outcomes is met with moral revulsion, because it seems to suggest an inflexibility to them—and that there is nothing we can do to help those less fortunate.
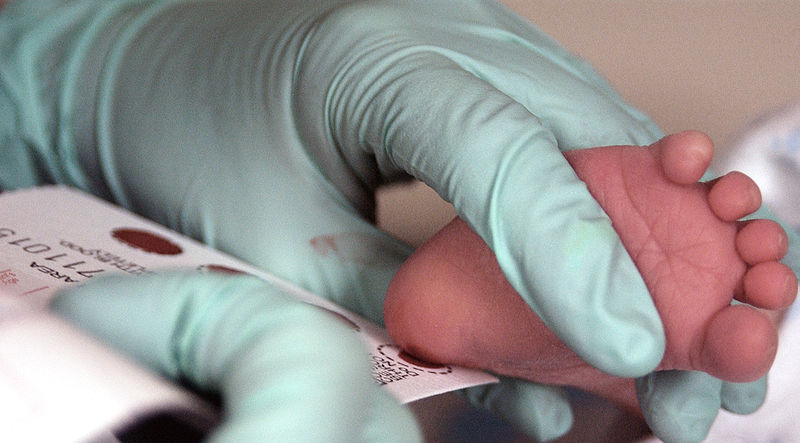
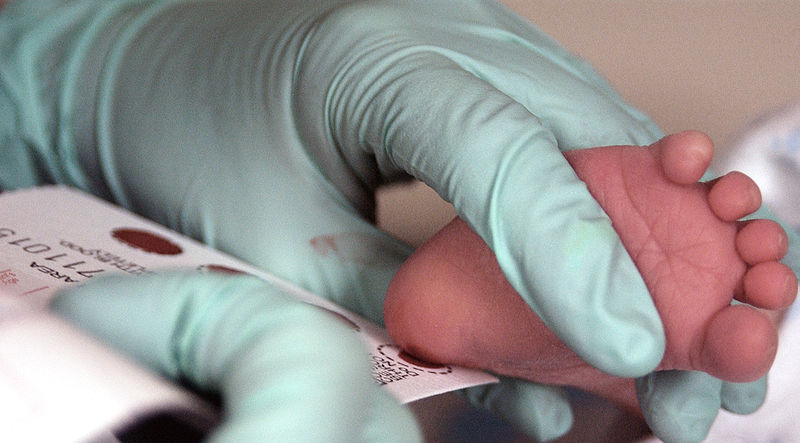
But genetic factors do not operate in a vacuum; the environment can heavily influence how genes evolve and are expressed. Consider phenylketonuria, a genetic disorder that often results in cognitive deficits due to the buildup of amino acids that cannot be processed by the body. With a proper diet, however, a person can avoid the offending compound and thus suffer less impairment later in life. This simple method of altering the environment to ameliorate IQ-depressing factors would not have been possible without first understanding the relevant biological mechanism.
As in the causal chain between the genes “for” nest building and the shaping of the nest, more influence from a volatile environment creeps in at higher levels of organization. And if we have any hope at all of addressing the discrimination, poverty, and lack of opportunity that may exist systemically at the more complex levels in our own societies, we must have a good grasp of the more basic ones. Understanding the sources of differences in outcome offers the promise of raising all boats—improving quality of life and education for everyone.
And as the influence of genes on behavior becomes better understood, so too does the influence of “environment”—which is not a waste bin of hand-waving explanations, but a collection of measurable patterns of behavior which should be subjected to the lens of empiricism like anything else. We now know, for instance, that while parenting style has a near-zero influence on a child’s personality when grown, early social experiences, especially around puberty, can influence social status later in life. Parents can’t usually change our personalities, but our socialization in adolescence, which always includes the navigation of our roles in a group, maybe can, at least a little. And when parents can change our personality outcomes, it is not with private schools and music lessons, but with abuse and neglect.
Almost every measured trait that benefits society is partially heritable. We can and should work toward social equality, but we should not wish for lower heritability. Only in an unequal society can poverty, discrimination, and illness stand the chance of altering a person’s IQ from what it “should” be. In a healthy society, its only shaping force should be the growth of healthy neurons in a healthy brain—neurons that unfold from sets of instructions which are written differently in all of us. The source of this inequality is not societal injustice, but the living result of precious variability in the human genome.
High abilities in all areas through evolution—not just intelligence—are what ultimately produce a great deal of that which all cultures value most. And because we are highly social and cooperative animals, a strong dam and a beautiful nest can benefit everyone. We should celebrate, not malign, the fact that science is increasingly revealing some of those benefits to be woven into the physical reality of the human genome. These abilities bestowed on us by nature need all the nurture they can get.
Glossary
allele.
One of multiple versions of a gene, and a basic unit of heritable variation.
evolution.
The process by which different kinds of living organisms develop and diversify from earlier forms throughout the history of the earth.
genome.
The full suite of heritable genetic variants, consisting of DNA, of an organism.
genotype.
An organism’s particular genetic makeup that leads to a phenotype.
locus.
The specific position of a gene on a chromosome, relative to the locations of other genes on that chromosome.
nucleotide.
The subunit, or “building block”, of nucleic acids like DNA. Variation in nucleotide at a given position gives rise to phenotypic differences in a population.
phenotype.
The physical expression of an organism’s genetic makeup.
polygenic.
Influenced (as in a phenotypic trait) by many genes, rather than a single set of variants.
psychometric.
The scientific development and use of measurement methods for human traits and abilities.
Recommended Reading
References
Dawkins, R. (1982). The Extended Phenotype. Oxford University Press: Oxford.
Dawkins, R. (1997). In conversation with Richard Dawkins (interview).
Plomin et al. (2016) http://www.gwern.net/docs/genetics/2016-plomin.pdf
Trzaskowski et al. (2014) http://www.ncbi.nlm.nih.gov/pmc/articles/PMC3907681/
Klein et al. (2005) https://www.ncbi.nlm.nih.gov/pmc/articles/PMC1512523/
Rietveld et al. (2013) http://science.sciencemag.org/content/340/6139/1467
Rietveld et al. (2014b) https://static.usc.edu/documents/Psych%20Science%20-%20manuscript%20R2%20final.pdf
Okbay et al. (2016) http://www.nature.com/nature/journal/v533/n7604/full/nature17671.html
Rietveld et al. (2014a) http://www.pnas.org/content/111/38/13790.full
Harris, J. R. (1998). The Nurture Assumption. Free Press: New York.
Selzam et al. (2016) http://www.nature.com/mp/journal/vaop/ncurrent/full/mp2016107a.html























